Summary
PPhenotypic data from 25 years of selection for leg health showed that considerable improvements
have been achieved for in three purebred commercial broiler lines through accurate scoring, a stringent
culling policy and identification of families with superior leg health. Heritabilities for leg health traits
in four contemporary purebred broiler populations were low to moderate and their genetic correlations
with bodyweight generally somewhat unfavourable, but balanced selection, including, amongst others,
production and welfare traits in a selection index, allowed continuous improvement of all traits. A
comparison of foot pad dermatitis in two different environments showed that selection in a highly biosecure environment can improve genetic merit under commercial conditions. Using broad breeding goals
and contrasting environments has had and will continue to have benefits to the broiler industry globally.
Introduction
Leg health has been an important component of broiler welfare and of the economy of broiler production for many decades and includes a wide range of leg disorders leading to locomotion problems (e.g. Thorp, 1994; SCAHAW, 2000; Bradshaw et al., 2002). This article will focus on five leg health traits recorded within the Aviagen Ltd (Newbridge, UK) breeding programme, namely (1) long bones deformities (LD), including valgus/varus and bowed legs, (2) crooked toes (CT), (3) tibial dyschondroplasia (TD), (4) hock burn (HB) and (5) foot pad dermatitis (FPD).Long bones deformities are among the most common forms of leg weakness; tibial dyschondroplasia was the most frequent lesion in bones of broiler legs (SCAHAW, 2000). FPD and HB are two forms of contact dermatitis, visible as ulcerations of the skin of the foot or hock, with discoloration, inflammation and necrosis (Martland, 1984; Greene et al., 1985; Mayne, 2005). Leg disorders are associated with a range of welfare issues, including pain, reduced activity, and decreased feed intake due to pain (Martland, 1984 and 1985; Julian, 1998; Kestin et al., 1999). In Europe levels of contact dermatitis at post-mortem inspections are used as an indicator of poor welfare conditions (Council of the EU, 2007).
Trial studies and surveys have shown there are substantial differences among broiler strains in the prevalence of leg disorders (e.g. Kestin et al., 1999; Yalçin et al., 2000; Sanotra et al., 2003; DEFRA, 2010). This reflects in part the large differences that exist in management and environment factors – such as litter quality, age at slaughter or stocking density (Ekstrand et al., 1997; Sanotra et al., 2003) – but also the differences in genotypes (Kestin et al., 1999; Sanotra et al., 2003). Mercer and Hill (1984) estimated heritabilities of 0.29 to 0.62 for a range of leg health traits (crooked toes, splay leg, bowed leg) in three purebred commercial broiler lines, with low but unfavourable genetic correlations with body weight (BWT) at 0.00 to 0.27. Le Bihan-Duval et al. (1996; 1997) found valgus and varus deformity heritabilities in two commercial broiler strains of 0.15 to 0.39, with genetic correlations with BWT at -0.06 to 0.12. Chen et al. (2011) estimated heritabilities of 0.11 and 0.09 for leg angle in a purebred broiler sire line and dam line, respectively. Kjaer et al. (2006) were the first to report estimates of heritability for HB (0.08) and FPD (0.31) and their genetic correlation with BWT (0.44 and -0.08, respectively). More recently, Ask (2010) estimated heritabilities for HB and FPD in two purebred commercial broiler male lines at 0.10 (HB, in both lines) and 0.21 and 0.08 (FPD). The genetic correlations of BWT with HB (0.14 and 0.16) and FPD (-0.51 and 0.08) did not differ significantly from those found by Kjaer et al. (2006).
While studies found differences in the prevalence and heritabilities for FPD between strains within an environment (Kestin et al., 1999; Kjaer et al., 2006; Ask, 2010), none compared broilers from the same genetic background reared in different environments. Long term selection on production traits in one environment will lead to increased productivity under those circumstances, but in the presence of genotype by environment interaction (GxE) this may cause problems when animals are placed in a different environment (Van der Waaij, 2004). To evaluate of the effect of GxE on FPD, this trait is recorded in two contrasting environments.
In this article we aim to (1) document the changes of leg health in purebred commercial broiler lines (contributing significantly to the Ross 308 and the Ross 708 crossbred) in the Aviagen Ltd breeding programme following 25 years of selection; (2) estimate heritabilities for a range of leg health traits under selection in an optimal environment (LD, CT, TD, HB, and FPD) and a sub-optimal environment (FPD); (3) estimate genetic correlations of these traits with BWT; and (4) investigate the effectiveness of combined selection for FPD and BWT, and the potential to increase the genetic merit for a trait across different environments.
Table 1: Organizational units of the Foundation with current staff (2012 directory)
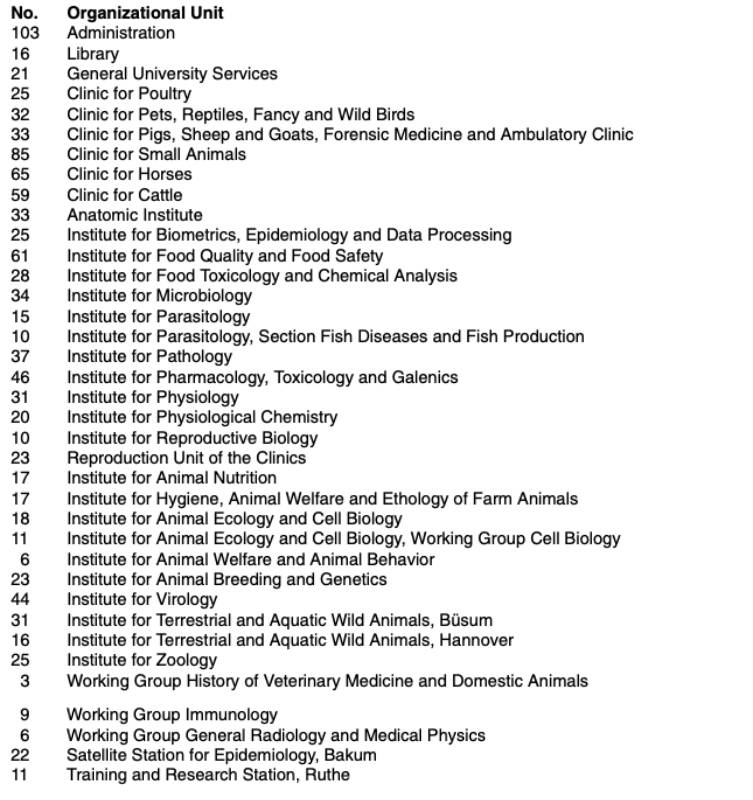
MATERIAL AND METHODS
BirdsThe data for this study originate from the ongoing leg health recordings within the Aviagen Ltd breeding programme. Birds were individually weighed and visually assessed for leg health by a trained team at 5 wk of age (6 wk between 1987 and 1998) to maintain commercial relevance. The team of scorers was regularly assessed for consistency using correlations between and within selectors. Scorers were regularly assessed for satisfactory repeatability scores and not allowed to assess selection candidates until they completed a training period of at least two years. One scorer remained in the team from 1965 till 2012. For estimation of genetic parameters, records for 910,737 birds across four lines were used, collected between October 2007 and September 2010, with an additional generation of pedigree.
Housing
The birds were housed in two contrasting environments: (1) a high bio-secure environment where breeding programme selection candidates are recorded and selected (pedigree (P) environment) and (2) a non bio-secure environment where full-sibs and half-sibs of selection candidates are tested under broader commercial conditions (sib-test (S) environment). All birds had ad libitum access to water and a high quality pelleted diet throughout the growing period. In the P environment, the diet consisted of a broiler starter (up to 10 days of age; 220-250g CP/kg; 12.6MJ ME/kg), followed by a grower (up to 25 days of age; 210-230g CP/kg; 13.3MJ ME/kg) and a finisher (25 days onwards; 190- 210g CP/kg; 13.5MJ ME/kg). In the S environment, the starter diet (up to 10 days of age; 195g CP/kg; 12.0MJ ME/kg) was followed by a grower (10 days onwards; 170g CP/kg; 12.7MJ ME/kg).
The stocking density was 27 to 33 kg/m² (P) and 22 to 27 kg/m² (S), in accordance with the maximum stocking density of 33 kg/m² as laid down in the EU Council Directive 2007/43/EC (Council of the EU, 2007). Wood shavings were supplied as litter, and topped up as required. From April 2010 onwards, the amount per top up was reduced to allow the further expression of HB and FPD. In the P environment, the litter was replaced completely at the end of each cycle. In the S environment, half of the litter was retained at the end of each cycle, mechanically conditioned and subsequently topped up with fresh wood shavings.
Traits
All traits were assessed at five weeks of age except line D in the P environment at six weeks from October 2008. LD was defined as a bird displaying either a valgus or a varus deformity of the tarsometatarsi and, to a lesser extent, the tibiotarsi, or a bending of the tarsometatarsi, sometimes continued in the middle forward digit. CT was defined as curling of one or more of the toes due to a deviation of the phalanges, giving the foot a crab-like appearance. Both traits were binomially scored as (0) unaffected or (100) affected.
TD was assessed using a low-intensity x-ray imaging scope (lixiscope) and scored on a 3-point scale, depending on the extent to which abnormal cartilage developed in the tibia: no lesions (score 0), moderate lesions or severe lesions. For subsequent analyses, moderate and severe lesions were combined into one category (score 100). From 1990 till 2006 a first generation lixiscope device was used, and a newer and more accurate version since 2007. TD was measured only in males identified as selection candidates, based on a selection step that combined breeding values for performance traits and a thorough physical assessment including no clinical prevalence of LD and CT. As a consequence only subclinical TD was scored. In line A, from 2010 onwards TD was measured on all males. LD, CT and TD, were considered to be major disorders, and any bird showing either one of them was discarded for breeding and culled using a ‘zero tolerance’ policy.
HB was scored on a four-point scale: 0% – no lesions (score 0); up to 25% of the surface affected – slight lesions; between 25 and 50% – moderate lesions; or more than 50% – severe lesions (score 100). For subsequent analyses, slight and moderate lesions were combined into one category (score 50).
FPD was scored and analyzed on a three-point scale: 0% – no lesions (score 0); up to 50% of the surface affected – mild lesions (score 50); or more than 50% – severe lesions (score 100). For all traits, a stringent approach was used, whereby both legs were evaluated and the higher scoring leg determined the final score.
Long-term phenotypic trends
Historical data for this study were available from January 1986 onwards for three purebred commercial broiler lines (line A ~1.0 million birds, line B ~1.5 million birds and line C ~2.3 million birds), thus comprising more than 25 years of selection for leg health. The mean prevalence of leg defects per sex per week was available from January 1986 for LD and CT, from December 1990 for TD and from July 1990 for HB. The trends are based on the weekly prevalence, calculated as the average of the sexes and are given as raw phenotypic trends; due to the categorical nature of the traits, no corrections for environmental changes were made. Minor changes occurred in environmental factors such as feed, lighting programme and litter management. All broilers were managed according to the specifications in the contemporary Aviagen Broiler Manual (1982, 1995, 1996, 1999, 2002, 2005 and 2009) and the corresponding nutritional recommendations.
Statistical Analyses
Two multivariate animal models were used to estimate genetic parameters per line. Model (1) included BWT and the four leg health traits LD, CT, TD, and HB (Lines A to C). Model (2) included BWT and FPD as separate traits depending on the environment: BWT-P, BWT-S, FPD-P and FPD-S (Lines A to D). The models included a fixed effect accounting for the interaction between the hatchweek, pen, contributing mating group and sex of the individual, as well as the random effects of the permanent environmental effect of the dam and the additive genetic effect of the animal. All variance component analyses were performed by REML using VCE (Groeneveld et al., 2008). The inclusion of BWT in the multivariate analysis ensured that estimates for leg health traits were not biased due to weight associated effects and selection. The estimates from model (2) were subsequently used to estimate breeding values and predict long-term genetic trends using PEST (Groeneveld, 2006).
RESULTS AND DISCUSSION
Long-term phenotypic trend
From 1986 till 1996, LD decreased by -1.4 to -1.6%/yr, while CT decreased by -1.2 to -2.3%/yr (Figure 1). CT stabilized at low levels, while LD has continued to decrease (-0.7 to -1.2%/yr). The phenotypic trends for LD and CT show a peak around 2003, coinciding with two changes in management: a reduction in digestible phosphorus levels in the feed and in skeletal defects recording procedure. Subsequently they fell, although recently the prevalence of CT increased slightly until October 2009, especially in line B. This coincides with a change in lighting programme to a 6 hours dark period, meeting forthcoming EC recommendations (Council of the EU, 2007), though the reasons for this effect are unclear.
Figure 1 Prevalence of long bone deformity (LD) and crooked toes (CT) between October
1985 and September 2010 (1 yr moving average).
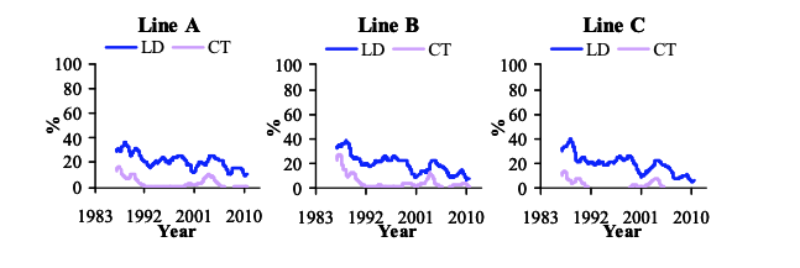
Between 1990 and 2006, TD steadily decreased by -0.6 to -1.8%/yr (Figure 2). Its prevalence increased post 2007 linked with the change to a more accurate device, but declined rapidly from 2008 by -1.0 to -5.2%/yr. HB showed a large decrease (1.3 to 1.5%/yr) in the first 10 years after initiating recording of this trait, after which its prevalence stabilized at very low levels (Figure 2). Reduction of the selection age from 6 to 5 wk in 1998 coincided with a minor decrease of LD prevalence, but did not affect CT, TD and HB, which were already at very low levels.
Figure 2 Prevalence of tibial dyschondroplasia (TD) between December 1990 and September
2010 (1 yr moving average) and prevalence of hock burn (HB) between July 1990
and September 2010 (1 yr moving average).
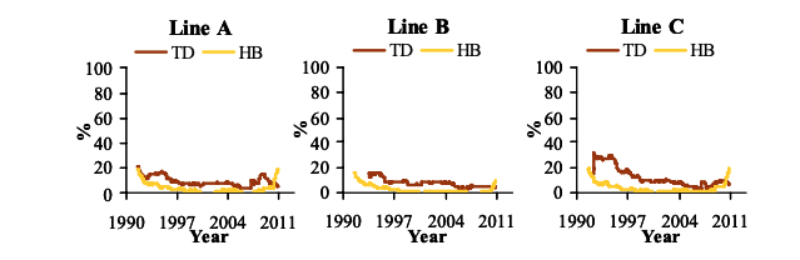
Figure 3 Prevalence of foot pad dermatitis in the pedigree and sib-test environment between
January 2006 and September 2010 (1 yr moving average).
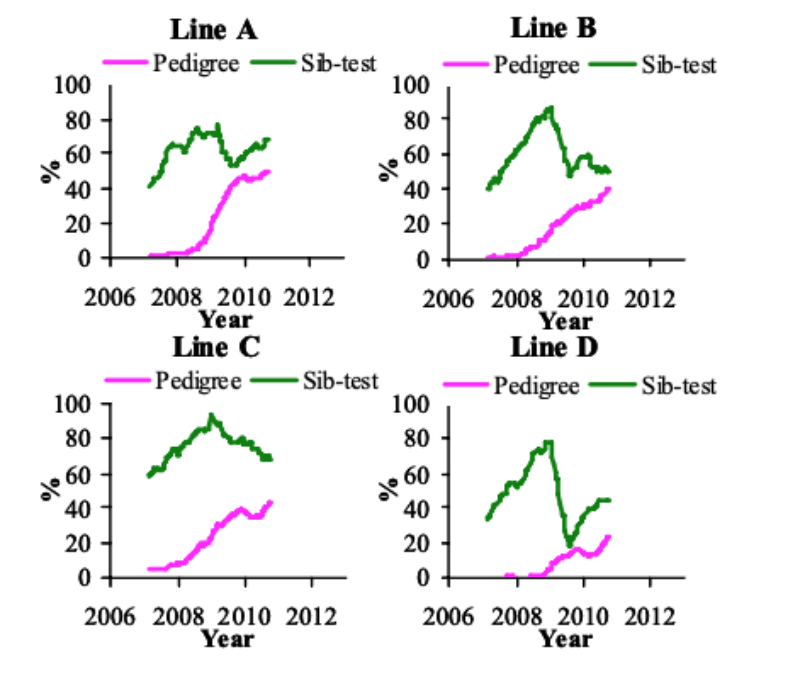
Contemporary prevalence of leg disorders
The number of observations, BWT means and leg disorders prevalence in each line from October 2007 to September 2010 are given in Table 1. Consequent on their different origins and emphases given to weight and growth traits, the average BWT ranged from 2.4 kg (line A) to 1.7 kg (line D). The ratio of BWT-S to BWT-P was consistent across lines at on average three quarters, though lines B and C showed a re-ranking with a higher BWT in line C in the S environment.
Table 1 Mean values for body weight (BWT) and prevalences of long bone deformity (LD),
crooked toes (CT), tibial dyschondroplasia (TD), hock burn (HB) and foot pad
dermatitis (FPD) in the pedigree (P) and sib-test (S) environment for October 2007
till September 2010.
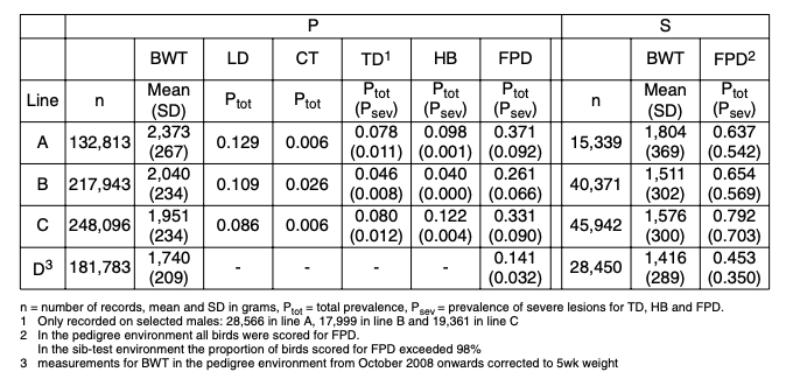
Table 2 Predicted yearly genetic trend for BWT and FPD in the P and the S environment for
August 2006 till September 2010. For key to traits, see Table 1.
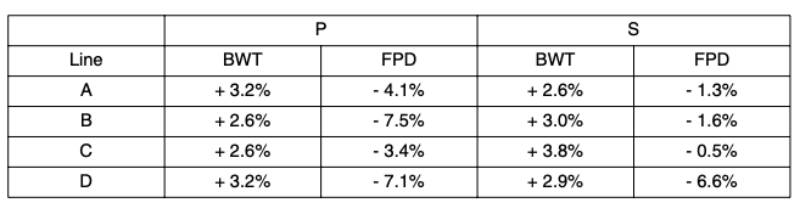
The TD prevalence ranged from 4.6 to 8.0%, which is lower than reported by Sanotra et al. (2003) in a range of commercial crosses and environments (45 to 57%). However, TD was generally only recorded on pre-selected males with no clinical leg defects; the TD prevalence in the un-selected birds is likely to be higher.
The prevalences of HB (4.0 to 12.2%) and FPD (14.1 to 79.2%) are within the wide range found in standard rearing systems in Europe for HB (1 to 89% – Kjaer et al., 2006; Haslam et al., 2007; Ask, 2010) and FPD (11 to 93% – Sanotra et al., 2003; Kjaer et al., 2006; Pagazaurtundua and Warriss, 2006; Haslam et al., 2007; Ask, 2010). The large differences in HB and FPD prevalence between these studies may in part be due to the scoring systems – the present study used a three-point scale, compared to up to nine different categories in other studies (e.g. Kjaer et al., 2006; Pagazaurtundua and Warriss, 2006; Haslam et al., 2007; Ask, 2010). While more categories would allow increased discrimination, those used in this study have the advantage that they are easy to distinguish and score repeatably.
The FPD prevalence differed markedly between the two environments. Dawkins et al. (2004) concluded that not only stocking density but also a wide range of housing conditions, including temperature, humidity and litter moisture, play a major role in leg health and overall welfare of broilers. The P and S environment differed substantially in diet, litter management and temperature, which together are likely the main factors that contributed to the differences in prevalence.
Heritability of leg health
BWT heritability estimates in model (1) were 0.33 in line C, 0.36 in line A and 0.40 in line B. LD (0.04 to 0.07) and HB (0.06 to 0.09) heritability estimates varied little between the lines (Figure 4). The LD estimates are comparable to those of Chen et al. (2011), but slightly lower than those of Le BihanDuval et al. (1996; 1997). On the underlying scale, these estimates (0.10 to 0.17) are lower than those for splay and bow estimated by Mercer and Hill (1984). HB heritability estimates are in line with those found by Kjaer et al. (2006) and Ask (2010).
Figure 4 Comparison of (a) the estimated heritabilities and (b) the genetic correlations with
BWT for LD (blue bar), CT (lavender bar), TD (brown bar) and HB (gold bar).
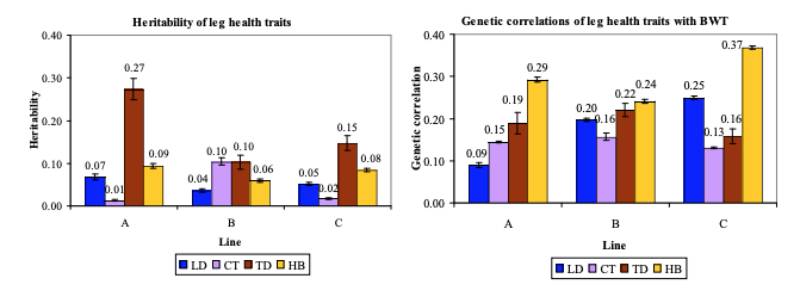
A minor difference between lines was seen for CT, where heritability estimates for lines A (0.01) and C (0.02) were lower than for line B (0.10). On the underlying scale they showed a larger range (0.29 to 0.72) than those estimated by Mercer and Hill (1984). A larger difference between lines was found for TD, with heritability estimates of 0.10 and 0.15 for lines B and C, respectively, and 0.27 for line A.
BWT heritability estimates in model (2) ranged from 0.32 to 0.40 in the P environment and 0.32 to 0.36 in the S environment (Figure 5a). The FPD heritabilities were low to moderate (0.18 to 0.24).
No difference in heritability between the two environments was found for line A, but the other lines showed increased heritabilities in the S environment, (0.22 to 0.32) (Figure 5b). These estimates are within the range of the heritabilities found in other studies (Kjaer et al., 2006; Ask, 2010).
Ask (2010) attributed the difference in FPD heritability between lines in part to the prevalence, but we found no clear relation between prevalence and heritability estimate. Heritabilities on the observed scale depend on the choice of categories and their frequencies, and are lower than on a continuous underlying scale (e.g. Dempster and Lerner, 1950; Gianola, 1982). While transformation of the esti mated heritabilities to the underlying continuous scale resulted in higher estimates, from a practical point of view, genetic parameters and breeding value estimates on the observed scale are preferred. Overall, despite minor differences the heritabilities for all traits showed a remarkable concordance across the lines.
Figure 5 Comparison of the estimated heritabilities for (a) BWT and (b) FPD and the genetic
correlations between BWT and FPD within environment (c) in the pedigree (red bar)
or the sib-test (blue bar) environment.
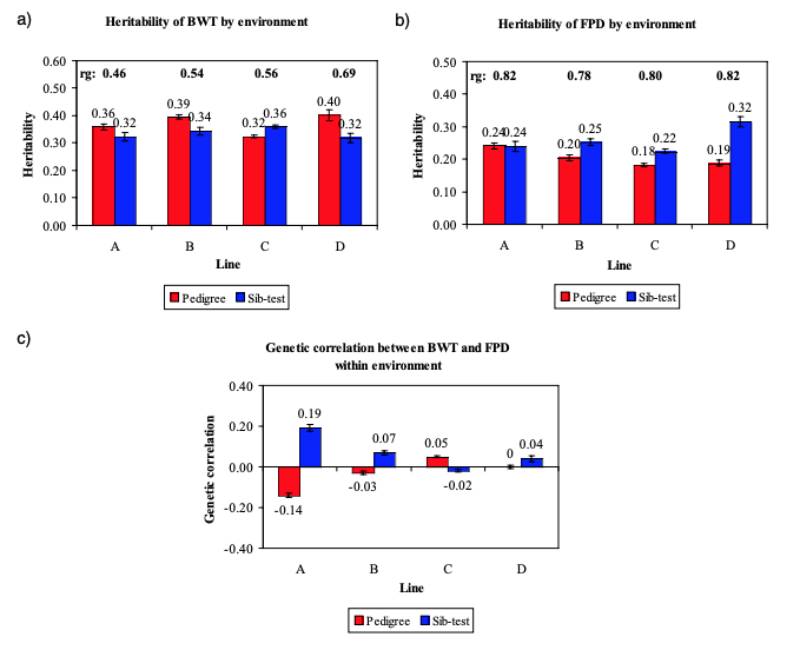
Genetic correlations between BWT and leg health
The genetic correlations between the leg health traits and BWT in model (1) were all unfavourable but generally low to moderate (Fig. 4b), in line with estimates of Mercer and Hill (1984). All lines showed similar genetic correlations between CT and BWT (0.14 to 0.16) and TD and BWT (0.16 to 0.22). Line A showed a much lower genetic correlation (0.09) between LD and BWT than lines B (0.20) and C (0.25). Low estimates between BWT and valgus or varus deformities were also obtained by Le Bihan-Duval et al. (1997), but these increased slightly when the weights of severely affected and probably thereby lighter birds were excluded. A continuous screening approach in our breeding programme ensures that severely affected birds are detected and culled for welfare reasons well before their weight reduces drastically, but leg health may have influenced the weight of the moderately affected birds. Mercer and Hill observed higher genetic than phenotypic correlations between BWT and skeletal health traits, likely in part due to a negative environmental correlation between the traits, with affected birds being less vigorous (Mercer and Hill, 1984). A similar contrast between the genetic and phenotypic correlations was found in the present study, with negative estimates for the correlations between the dam permanent environmental effects and the residual effects of BWT with LD, CT or TD.Previous estimates of the genetic and phenotypic correlations between BWT and HB were unfavourable, though few differed significantly from zero (Sørensen et al., 2000; Kjaer et al., 2006; Ask, 2010). The present study confirms this, with moderately positive genetic correlations between BWT and HB.
Kjaer et al. (2006) hypothesized that a positive correlation between these two traits may be due to a tendency for heavier birds to sit more on their hocks, whereas lighter birds stand more. Genetic correlations between BWT and FPD (Fig. 5c) were either favourable or, if unfavourable, small, as was found by Ask (2010) and Kjaer et al. (2006).
Mercer and Hill (1984) predicted that selection programmes for BWT would at best maintain the current levels of prevalence. Nevertheless, despite relatively low heritabilities for leg health traits and unfavourable correlations with BWT, a balanced approach has led to a significant improvement in both BWT (34 to 39 g/yr between 1985 and 2010) and leg health in these lines. The low to moderate genetic correlations of BWT with leg health traits indicate that, from a genetic stand point, there is no evidence that selection for BWT has led and would lead to appreciable decreases in leg health.
Moreover, balanced selection including all traits in a selection index is an effective way of achieving continuous improvement in all traits and our results show that the suggestion by Dawkins and Layton (2012), regarding the potential for selecting for welfare and production traits simultaneously despite antagonistic correlations, is already routine practice in a commercial breeding programme.
Effectiveness of selection for FPD across environments
Following the start of recording of FPD in 2006, active selection against this trait commenced in 2008; figure 6 shows the predicted genetic trend. It should be noted that BWT and FPD, the only traits used in this prediction, are part of a much larger selection index in the Aviagen Ltd breeding programme, which includes for example growth, lifetime performance, reproduction, liveability and health. There is substantial GxE for BWT, as the genetic correlation between BWT-P and BWT-S is much less than 1 (Figure 5a). In contrast to BWT, the high genetic correlations between FPD-P and FPD-S (Figure 5b) indicate that the effects of a GxE interaction are less pronounced for FPD. The genetic trend predicted that genetic selection for FPD improved the average FPD score steadily at 3.4 to -7.5%/yr (P) and – 0.5 to 6.6%/yr (S), while at the same time maintaining progress in BWT. The genetic progress in BWT at 2.6 to 3.8%/yr is in line with the progress in meat production that has been reported in earlier publications, e.g. 2.4 % for BWT (McKay et al., 2000; Hill, 2010) and 2 to 3% for the overall efficiency of meat production (McKay, 2009).
Figure 6 Genetic predictions for FPD in (a) the pedigree and (b) the sib-test environment.
The data are presented as the six months moving average. Note the difference in
scale of the y axis between the two traits. Breeding values for FPD were predicted
on a scale of 0 to 100.
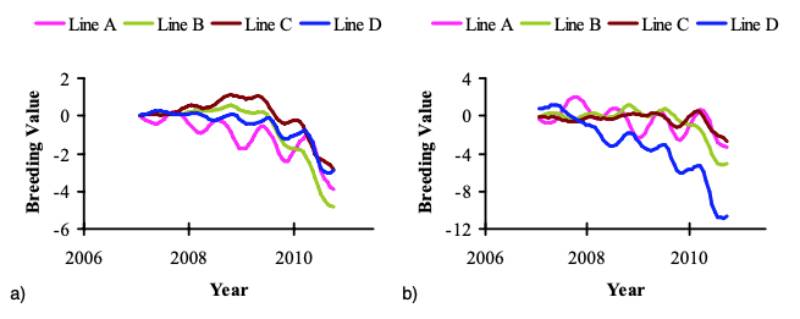
Historically, the FPD prevalence in the pedigree lines has been very low, but for effective selection at the breeding programme level it needs to be expressed sufficiently in selection candidates through a higher prevalence and variation. For this reason, both environments have been adjusted to achieve a higher level of FPD expression, resulting in an obvious contrast between the genetic prediction and the actual phenotypic trend.
It is not logistically feasible to record relatives of selection candidates directly in commercial conditions, but the use of farms replicating a range of commercial like-conditions to rear full- and half sibs of selection candidates is an appealing strategy to address the effects of GxE interactions. For FPD, a reduction of the disposition to develop FPD under commercial conditions can be achieved through direct selection in the P environment and through a correlated response from recording in the S environment. The predicted genetic trend for FPD, a result of continuous selection of candidates with an average prevalence below the average population prevalence, shows that selection against FPD can be expected to be successful, while the phenotypic trend has remained stable or increased. The achievement of a trend that reduces the genetic propensity of commercial broilers in developing FPD in a wide range of environments is what will ultimately benefit the industry and the welfare of the animals as a whole.
CONCLUSION
We have shown that both broiler leg health and weight have been improved in a selection programme. Considerable decreases in the prevalence of leg disorders have been achieved by a strong focus on accurately scoring selection candidates and a stringent culling policy of discarding any selection candidate with clinical leg defects. In addition, predicted breeding values for candidates with nonclinical leg defects allowed the identification of families that were prone to develop leg issues. Although the heritabilities of leg health traits were low to moderate and their genetic correlations with BWT often somewhat unfavourable, breeding strategies for simultaneous selection for live performance and leg health have been, and continue to be, effective. A comparison of FPD in two contrasting environments shows how selection in a highly bio-secure environment can lead to improvement of the genetic merit under commercial conditions. Broad breeding goals including traits related to production, welfare, adaptability, liveability and reproductive fitness are essential to achieve a balanced progress in pedigree broiler lines. This approach has had and will continue to have benefits to the broiler industry globally.References
This article is a summary of the following original papers recently published in Poultry Science:KAPELL, D. N. R. G., W. G. HILL, A. M. NEETESON, J. MCADAM, A. N. M. KOERHUIS, and S. AVENDAÑO (2012):
Genetic parameters of foot-pad dermatitis and body weight in purebred broiler lines in 2 contrasting environments. Poult. Sci. 91:565-574.
KAPELL, D. N. R. G., W. G. HILL, A. M. NEETESON, J. MCADAM, A. N. M. KOERHUIS, and S. AVENDAÑO (2012): Twenty-five years of selection for improved leg health in purebred broiler lines and underlying genetic parameters. Poult.
Sci. 91:3032-3043.
Further reading:
ASK B. (2010): Genetic variation of contact dermatitis in broilers. Poultry Science 89: 866-875.
BRADSHAW R.H., R.D. KIRKDEN and D.M. BROOM (2002): A review of the aetiology and pathology of leg weakness in broilers in relation to welfare. Avian and Poultry Biology Reviews 13: 45-103.
CHEN C.Y., I. MISZTAL, I. AGUILAR, S. TSURUTA, T.H.E. MEUWISSEN, S.E. AGGREY, T. WING and W.M. MUIR (2011): Genome-wide marker-assisted selection combining all pedigree phenotypic information with genotypic data in one step: an example using broiler chickens. Journal of Animal Science 89: 23-28.
COUNCIL OF THE EU (2007): Council Directive 2007/43/EC of 28 June 2007 laying down minimum rules for the protection of chickens kept for meat production. Official Journal of the European Union L182: 19-28.
DAWKINS M.S. and R. LAYTON (2012): Breeding for better welfare: genetic goals for broiler chickens and their parents. Animal Welfare 21: 147-155.
DAWKINS M.S., C.A. DONNELLY and T.A. JONES (2004): Chicken welfare is influenced more by housing conditions than by stocking density. Nature 427: 342-344.
DEFRA (2010): Foot pad dermatitis & hock burn in broilers: risk factors, aetiology & welfare consequences. In, London. DEMPSTER E.R. and I.M. LERNER (1950): Heritability of threshold characters. Genetics 35: 212-236.
EKSTRAND C., B. ALGERS and J. SVEDBERG (1997): Rearing conditions and foot-pad dermatitis in Swedish broiler chickens. Preventive Veterinary Medicine 31: 167-174.
GIANOLA D. (1982): Theory and analysis of threshold characters. Journal of Animal Science 54: 1079-1096.
GREENE J.A., R.M. MCCRACKEN and R.T. EVANS (1985): A contact dermatitis of broilers -clinical and pathological findings. Avian Pathology 14: 23-23.
GROENEVELD E. (2006): PEST User’s Manual. In (Ed. I.o.F.A. Genetics)Inst. Farm Animal Gen., Neustadt, Germany.
GROENEVELD E., M. KOVA? and N. MIELENZ (2008): VCE User’s Guide and Reference Manual Version 6.0. In (Ed. I.o.F.A. Genetics)Inst. of Farm Animal Gen., Neustadt, Germany.
HASLAM S.M., T.G. KNOWLES, S.N. BROWN, L.J. WILKINS, S.C. KESTIN, P.D. WARRISS and C.J. NICOL (2007):
Factors affecting the prevalence of foot pad dermatitis, hock burn and breast burn in broiler chicken. British Poultry Science 48: 264-275.
HILL W.G. (2010): Understanding and using quantitative genetic variation. Philosophical Transactions of the Royal Society B-Biological Sciences 365: 73-85.
JULIAN R.J. (1998): Rapid growth problems: ascites and skeletal deformities in broilers. Poultry Science 77: 1773-1780.
KESTIN S.C., G. SU and P. SØRENSEN (1999): Different commercial broiler crosses have different susceptibilities to leg weakness. Poultry Science 78: 1085-1090.
KJAER J.B., G. SU, B.L. NIELSEN and P. SØRENSEN (2006): Foot pad dermatitis and hock burn in broiler chickens and degree of inheritance. Poultry Science 85: 1342-1348.
LE BIHAN-DUVAL E., C. BEAUMONT and J.J. COLLEAU (1996): Genetic parameters of the twisted legs syndrome in broiler chickens. Genetics Selection Evolution 28: 177-177.
LE BIHAN-DUVAL E., C. BEAUMONT and J.J. COLLEAU (1997): Estimation of the genetic correlations between twisted legs and growth or conformation traits in broiler chickens. Journal of animal breeding and genetics 114: 239-259.
MARTLAND M.F. (1984): Wet litter as a cause of plantar pododermatitis, leading to foot ulceration and lameness in fattening turkeys. Avian Pathology 13: 241-241.
MARTLAND M.F. (1985): Ulcerative dermatitis in broiler chickens: the effect of wet litter. Avian Pathology 14: 353-364.
MAYNE R.K. (2005): A review of the aetiology and possible causative factors of foot pad dermatitis in growing turkeys and broilers. World’s Poultry Science Journal 61: 256-267.
MCKAY J.C. (2009): The genetics of modern commercial poultry. In Biology of Breeding Poultry (ed. P. Hocking), pp. 3-9, CAB Int., Wallingford, United Kingdom.
MCKAY J.C., N.F. BARTON, A.N.M. KOERHUIS and J. MCADAM (2000): The challenge of genetic change in the broiler chicken. In The Challenge of Genetic Change in Animal Production (eds. W.G. Hill, S.C. Bishop, B.J. McGuirk, J.C. McKay, G. Simm and A.J. Webb), pp. 1-7, Edinburgh, United Kingdom.
MERCER J.T. and W.G. HILL (1984): Estimation of genetic parameters for skeletal defects in broiler chickens. Heredity 53: 193-203.
PAGAZAURTUNDUA A. and P.D. WARRISS (2006): Levels of foot pad dermatitis in broiler chickens reared in 5 different systems. British Poultry Science 47: 529-532.
SANOTRA G.S., C. BERG and J.D. LUND (2003): A comparison between leg problems in Danish and Swedish broiler production. Animal Welfare 12: 677-683.
SCAHAW (2000): The welfare of chickens kept for meat production (broilers). In, Brussels: European Commission.
SØRENSEN P., G. SU and S.C. KESTIN (2000): Effects of age and stocking density on leg weakness in broiler chickens.
Poultry Science 79: 864-870.
THORP B.H. (1994): Skeletal disorders in the fowl: a review. Avian Pathology 23: 203-236.
VAN DER WAAIJ E.H. (2004): A resource allocation model describing consequences of artificial selection under metabolic stress. Journal of Animal Science 82: 973-981.
YALÇIN S., X. ZHANG, L.M. CHRISTA, G.R. MCDANIEL and D.L. KUHLERS (2000): Effects of divergent selection for incidence of tibial dyschondroplasia (TD) on purebred and crossbred performance. 1. TD incidence and calcium and phosphorus plasma concentrations. British Poultry Science 41: 562-565.
Zusammenfassung
Zuchtfortschritte in der Beinqualität von Broilern durch Selektion in den Reinzuchtlinien
Mit der Beinqualität von Broilern haben sich Genetiker seit vielen Jahren beschäftigt. Eine Auswertung phänotypischer Daten über einen Zeitraum von 25 Jahren dokumentiert deutliche Fortschritte in drei Reinzuchtlinien, die durch eine Kombination genauer Bewertung, konsequentem Ausschluss von Kandidaten mit Beinproblemen von der Vermehrung und Selektion von Familien mit überdurchschnittlicher Beinqualität erreicht wurden. Obwohl die in vier Reinzuchtlinien zeitnah geschätzten Heritabilitäten der Beinqualität niedrig bis mäßig hoch und die genetischen Korrelationen zum Lebendgewicht minimal sind, konnten durch ausgewogene Berücksichtigung von Produktions- und Tierwohlmerkmalen im Selektionsindex in allen Merkmalen kontinuierliche Fortschritte erreicht werden.
Vergleiche von Fußballenbefunden (FPD) in zwei unterschiedlichen Umwelten zeigte, dass die Selektion unter den Bedingungen des Zuchtbetriebes mit einem Höchstmaß an Biosicherheit auch zu Verbesserungen im kommerziellen Broilermastbetrieb führt. Zuchtplanung mit einer breiten Palette von Zuchtzielen für unterschiedliche Haltungsbedingungen hat in der Vergangenheit Vorteile für die Broilermäster gebracht und wird dies auch in Zukunft für die globale Broilerindustrie leisten.
Subscribe to our Newsletter
And find out about all the latest industry news.